 |
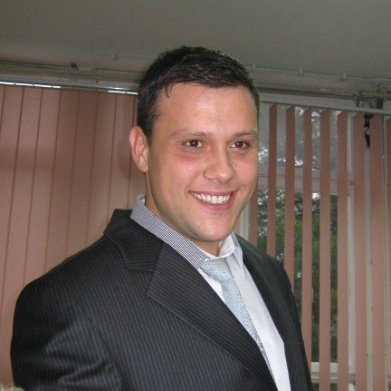
Vladimir Markovic, MPhys
Department of Physics,
Faculty of Sciences,
University of Kragujevac
34000 Kragujevac
Radoja Domanovica 12
Serbia
1. Printed Papers in Journals on International Scientific indexing list:
Papers are presented in reversed chronological order
1.14. Printed Paper:
Title: MCNP simulation of the dose distribution in liver cancer treatment for BNC therapy
Authors: Dragana Krstic, Zoran Jovanovic, Vladimir Markovic, Dragoslav Nikezic, Vlade Urosevic
Journal: Central European Journal of Physics
Print ISSN: 1895-1082
Online ISSN: 1644-3608
Volume: 12(10): 714-718, 2014, IF= 1.077 (M23)
doi: 10.2478/s11534-014-0507-2
Category of Journal in year of publication and previous two years:
Year 2014: IF= *****, (***)
Year 2013: IF= 1.077, (M23)
Year 2012: IF= 0.905, (M23)
Abstract
The Boron Neutron Capture Therapy (BNCT) is based on selective uptake of boron in tumour tissue compared to the surrounding normal tissue. Infusion of compounds with boron is followed by irradiation with neutrons. Neutron capture on 10B, which gives rise to an alpha particle and recoiled 7Li ion, enables the therapeutic dose to be delivered to tumour tissue while healthy tissue can be spared. Here, therapeutic abilities of BNCT were studied for possible treatment of liver cancer using thermal and epithermal neutron beam. For neutron transport MCNP software was used and doses in organs of interest in ORNL phantom were evaluated. Phantom organs were filled with voxels in order to obtain depth-dose distributions in them. The result suggests that BNCT using an epithermal neutron beam could be applied for liver cancer treatment.
References
[1] D. L. Bleuel, W. T. Chu, R. J. Donahue, B. A. Ludewigta, J. Vujic, Med. Phys. 25, 1725 (1998)
[2] F. El Moussaoui, T. El Bardouni, M. Azahra, A. Kamili, H. Boukhal, Cancer/Radiotherapie 12, 360 (2008)
[3] A. Zonta et al., Appl. Radiat. Isot. 67, S67 (2009)
[4] G. Gambarini, G. G. Daquino, R. L. Moss, M. Carrara, V. A. Nievaart, E. Vanossi, Radiat. Prot. Dosim. 126, 604 (2007)
[5] T. Pinelli et al., Eighth International Symposium, 1998, Los Angeles, CA, USA (Kluwer Academic/Plenum Publishers, New York, 2001) 1427
[6] A. Zonta et al., J. Phys.: Conference Series 41, 484 (2006)
[7] S. Bortolussi et al., Appl. Radiat. Isot. 69, 1924 (2011)
[8] L. Kankaanranta et al., Int. J. Radiation Oncol. Biol.Phys. 69, 475 (2007)
[9] I. Porras, Phys. Med. Biol. 57, L1 (2008)
[10] I. Porras, Appl. Radiat. Isot. 69, 1838 (2011)
[11] H. Horiguchi, T. Nakamura, H. Kumada, H. Yanagie, M. Suzuki, H. Sagawa, Appl. Radiat. Isot. 69, 1882 (2011)
[12] D. Krstic, V. M. Markovic, Z. Jovanovic, B. Milenkovic, D. Nikezic, J. Atanackovic, Radiat. Prot. Dosim. DOI:10.1093/rpd/nct365.
[13] K. F. Eckerman, M. Cristy, J. C. Ryman The ORNL Mathematical Phantom Series, Oak Ridge National Laboratory Report, (Oak Ridge, TN, USA, 1996) Updated 08 April (2009)
[14] D. Krstic, D. Nikezic, Comp. Phys. Commun. 176, 33 (2007)
[15] X-5 Monte Carlo Team, MCNP’a General Monte Carlo N-Particle Transport Code, Version 5 Vol. I: Overview and Theory (Los Alamos National Laboratory, Los Alamos, 2003)
[16] T. Matsumoto, Nucl. Instrum. Meth. A 580, 552 (2007)
1.13. Printed Paper:
Title: Monte carlo calculations of lung dose in ORNL phantom for boron neutron capture therapy
Authors: D. Krstic, V.M. Markovic, Z. Jovanovic, B. Milenkovic, D. Nikezic, J. Atanackovic
Journal: Radiation Protection Dosimetry
ISSN: 0144-8420
Volume 161(1-4): 269-273, 2014, IF= 0.909 (M22)
doi: 10.1093/rpd/nct365
Category of Journal in year of publication and previous two years:
Year 2014: IF= *****, (***)
Year 2013: IF= 0.861, (M22)
Year 2012: IF= 0.909, (M23)
Abstract
Monte Carlo simulations were performed to evaluate dose for possible treatment of cancers by boron neutron capture therapy (BNCT). The computational model of male ORNL phantom was used to simulate tumors in the lungs. Calculations have been performed by means of the MCNP5/X code. In this simulation two opposite neutron beams were considered, in order to obtain uniform neutron flux distribution inside the lungs. The obtained results indicate that the lung cancer could be treated by BNCT under the assumptions of calculations.
References
- Farr, Le; Sweet, Wh; Robertson, Js; Foster, Cg; Locksley, Hb; Sutherland, Dl; Mendelsohn, Ml; Stickley, Ee (1954). "Neutron capture therapy with boron in the treatment of glioblastoma multiforme". the american journal of roentgenology, radium therapy, and nuclear medicine 71 (2): 279–93.
- Yasui, L., Kroc, T., Gladden, S., Andorf, C., Bux, S. and Hosmane, N. Boron neutron capture in prostate cancer cells. Appl. Radiat. Isot. 70, 6–12 (2012).
- Hopewell, J.W., Morris, G.M., Schwint, A. and Codere, J.A. The radiobiological principles of boron neutron capture therapy: A critical review. Appl. Radiat. Isot. 69, 1756-1759 (2011).
- Voyant, C., Roustit, R., Tatje, J., Biffi, K., Leschi, D., Briançon, J. and Lantieri Markovici, C. Therapeutic potential of atmospheric neutrons. Rep. Pract. Oncol. Radiother. 16, 21-31 (2011).
- Altieri, S., Bortolussi, S., Bruschi, P., Fossati, F., Vittor, K., Nano, R., Facoetti, A., Chiari, P., Bakeine, J.G., Clerici, A., Ferrari, C. and Salvucci, O. Boron absorption imaging in rat lung colon adenocarcinoma metastases. J. Phys.: Conf. Series 41, 484–495 (2006).
- Bortolussi, S. and Altieri, S. Thermal neutron irradiation field design for boron neutron capture therapy of human explanted liver. Med. Phys. 34, 4700-4705 (2007).
- Protti, N., Bortolussi, S., Stella, S., Gadan, M. A., De Bari, A., Ballarini, F., Bruschi, P., Ferrari, C., Clerici, A. M., Zonta, C., Bakeine, J. G., Dionigi, P., Zonta, A. and Altieri, S. Calculation of dose distribution in the lung of a rat model irradiated in the thermal column of the TRIGA reactor in Pavia. Appl. Radiat. Isot. 67, 210-213 (2009).
- Verbeke J. M., Vujic, J. and Leung, K-N. Neutron beam optimization for Boron Neutron Capture Therapy using the D-D and D-T high-energy neutron sources. Nucl. Technol. 129, 257-258 (2000).
- Baba, H., Onizuka, Y., Nakao, M., Fukahori, M., Sato, T., Sakurai, Y., Tanaka, H. and Endo, S. Microdosimetric evaluation of the neutron field for BNCT at Kyoto University reactor by using the PHITS code. Radiat. Prot. Dosim. 148, 528-532 (2011).
- Blaickner, M., Kratz, J.V., Minouchehr, S., Otto, G, Schmidberger, H., Schütz, C., Vogtländer L., B. Wortmann, B. and Hampel, G. Dosimetric feasibility study for an extracorporeal BNCT application on liver metastases at the TRIGA Mainz. Appl. Radiat. Isot. 70, 139-143 (2012).
- Matsumoto, T. Monte Carlo simulation of depth–dose distribution in several organic models for boron neutron capture therapy. Nucl. Instrum. Meth. A 580, 552-557 (2007).
- X-5 Monte Carlo Team. MCNP–a General Monte Carlo N-Particle Transport Code, Version 5 Vol. I: Overview and Theory. Los Alamos, NM: Los Alamos National Laboratory; LA- UR- 03- 1987; (2003).
- Eckerman, K.F., Cristy, M. and Ryman, J.C. The ORNL mathematical phantom series. Oak Ridge National Laboratory. Oak Ridge, TN 37831, USA; 1996. Updated 08 April (2009).http://ordose.ornl.gov/resources/Mird.pdf.
- Bortolussi S, Boron Neutron Capture Therapy of disseminated tumours, Scientifica Acta 1, No. 1, 123 – 128 (2007)
- Krstic, D. and Nikezic, D. Input files with ORNL-mathematical phantoms of the human body for MCNP-4B. Comp. Phys. Commun. 176, 33-37 (2007).
- Goorley T. MCNP Medical Physics Geometry Database. Los Alamos National Laboratory. http://mcnp-green.lanl.gov/publication/pdf/MP_DataBase_March2008-LA-UR-08-2113-release.pdf Also available on http://www.pmf.kg.ac.rs/radijacionafizika/Products.html
- Neutron Fluence Measurements, IAEA Technical Reports Series No. 107, International Atomic Energy Agency, 1970.
1.12. Printed Paper:
Title: Specific energy distribution within cytoplasm and nucleoplasm of a typical mammalian cell due to various beta radionuclides
Authors: V.M. Markovic, N. Stevanovic, D. Nikezic, Dž. F. Pucic, V. Urosevic
Journal: Journal of Radioanalytical and Nuclear Chemistry
Print ISSN: 0236-5731
Online ISSN: 1588-2780
Volume: 299(3): 1723-1730, 2014, IF=1.467 (M21)
doi: 10.1007/s10967-013-2874-3
Category of Journal in year of publication and previous two years:
Year 2013: IF= 1.415, (M21)
Year 2012: IF= 1.467, (M21)
Year 2011: IF= 1.520, (M21)
Abstract
The use of beta radionuclides for treatment in radiotherapies leads for a need of better understanding of interactions and local energy depositions of beta particles within tissue and tissue equivalent media. The aim of this work is to determine microdosimetric quantities for various radionuclides. Specific energy, z, and its distribution, f(z), mean specific energy, z, and its standard deviations, σ(z), were evaluated for typical single cell where nucleus is the cellular region of interest. Three possible positions of readionuclides were taken into account – cellular membrane, cytoplasm and nucleoplasm. Taking these regions as the source of radiation, microdosimetric quantities were calculated for beta emitting radionuclides: 191Os, 199Au, 177Lu, 67Cu, 77As, 131I, 186Re, 32P, 188Re and 90Y. It was found that low range beta emitters have the largest efficiency and can deliver radiation doses up to 1 mGy per decay. Determination of the specific energy and its distribution for radionuclides is a useful start in order to determine beta emitters for a particular radionuclide therapy.
References
- Milenic DE, Brechbiel MW (2004) Targeting of radioisotopes for cancer therapy Cancer. Biol. Ther. 3:361-370
- Milenic DE, Brady ED, Brechbiel MW (2004) Antibody-targeted radiation cancer therapy Nature Rev Drug Discovery 3:488-98
- Bousis C, Emfietzoglou D, Hadjidoukas P, Nikjoo H (2010) Monte Carlo single-cell dosimetry of Auger-electron emitting radionuclides, Phys. Med. Biol. 55:2555–2572
- Bloch WE and Kim EH (1994) Calculations of electron single event distributions for use in internal beta microdosimetry Rad. Prot. Dos. 52:77-80
- Kaminski MS, Tuck M, Estes J, Kolstad A, Ross CW, Zasadny K, Regan D, Kison P, Fisher S, Kroll S, Wahl RL (2005) (131) I-Tositumomab therapy as initial treatment for follicular lymphoma N. Eng. J. Med. 353:441-449
- Ferro-Flores G, Torres-Garcia E, Garcia-Pedroza L, Arteaga-Perez C, Pedraza-Lopez M, Garnica-Garza HM (2005) An efficient, reproducible and fast preparation of 188Re-anti-CD20 for the treatment of non-Hodgkin lymphoma Nucl. Med. Commun. 26:793-799
- Syme AM, Kirkby C, Riauka TA, Fallone BG, McQuarrie SA (2004) Monte Carlo investigation of single cell beta dosimetry for intraperitoneal radionuclide therapy Phys. Med. Biol. 49:1959-1972
- Humm JL, Cobb LM (1990) Nonuniformityof tumor dose in radioimunotherapy J. Nucl. Med. 31:75-83
- Hindorf C, Emfietzoglou D, Lindén O, Bousis C, Fotopoulos A, Kostarelos K, Flux GD (2007) Single-Cell Dosimetry for Radioimmunotherapy of B-Cell Lymphoma Patients with Special Reference to Leukemic Spread Cancer Bioth. Radioph. 22(3):357-366
- Hindorf C, Emfietzoglou D, Lindén O, Kostarelos K, Strand SE (2005) Internal Microdosimetry for Single Cells in Radioimmunotherapy of B-Cell Lymphoma Cancer Bioth. & Rad. 20(2):224-231
- Kriehuber R, Riedling M, Symko M, Weiss DG (2004) Cytotoxicity, genotoxicity and intracellular distribution of the Auger electron emitter 65Zn in two human cell lines Radiat. Environ. Biophys. 43:15–22
- Neti PVSV, Howell RW (2006) Log normal distribution of cellular uptake of radioactivity: implications for biologic responses to radiopharmaceuticals J. Nucl. Med. 47:1049–58
- Nikjoo H (2003) Radiation track and DNA damage, Iran. J. Radiat. Res. 1(1): 3 – 16
- ICRU Report 36 (1983) Microdosimetry, Washington DC
- Kellerer A (1985) Fundaments of microdosimetry, The dosimetry of ionizing radiation, Academic press, INC, London.
- Liamsuwan T, Emfietzoglou D, Uehara S, Nikjoo H Microdosimetry of low-energy electrons Int. J. Radiat. Biol. 88(12):899-907
- Sgouros G (2005) Dosimetry of internal emitters J. Nucl. Med. 46:18S–27S
- Stabin M (2006) Nuclear medicine dosimetry Phys. Med. Biol. 51:R187–R202
- Ekström LP, Firestone RB (2013) WWW Table of Radioactive Isotopes, database version 2/28/99, [cited 2013 September 5]. Available from: http://ie.lbl.gov/toi/
- Salvat F, Ferna´ndez-Varea JM, Sempau J (2006) PENELOPE–2006 a code system for Monte Carlo simulation of electron and photon transport. OECD Nuclear Energy Agency, Issy-les-Moulineaux
- Baró J, Sempau J, Fernández-Varea JM, Salvat F (1995) PENELOPE: An algorithm for Monte Carlo simulation of the penetration and energy loss of electrons and positrons in matter Nucl. Instr. Meth. B 100(1):31–46
- Stewart RD, Wilson WE, McDonald JC and Strom DJ (2002) Microdosimetric properties of ionizing electrons in water: a test of the PENELOPE code system, Phys. Med. Biol. 47:79–88
- Fernández-Varea JM, González-Muñoz G, Galassi ME, Wiklund K, Lind BK, Ahnesjö A, Tilly N (2012) Limitations (and merits) of PENELOPE as a track-structure code Int. J. Radiat. Biol. 88(1–2): 66–70
- Salvat F, Fernandez-Varea JM, Baro J and Sempau J (1996) PENELOPE, An algorithm and computer code for Monte Carlo simulation of electron-photon showers, Informes Tecnicos CIEMAT no 799 Madrid
- Nikjoo H, Ueharab S, Emfietzoglouc S, Cucinottad EA (2006) Track-structure codes in radiation research Radiation Measurements 41:1052-1074
- Torres-Garcia E, Garnica-Garza HM, Ferro-Flores G, 2006 Monte Carlo microdosimetry 188Re- and 131I-labelled anti-CD2 Phys. Med. Biol. 51:N349-N356
- Semenenko VA, Turner JE, Borak TB (2003) NOREC, a Monte Carlo code for simulationg electron track in liquid water Radiat. Environ. Biophys. 42:213-217
- Friesen C, Lubatschofski A, Kotzerke J, Buchmann I, Reske SN, Debatin KM (2003) Beta-irradiation used for systemic radioimmunotherapy induces apoptosis and activates apoptosis pathways in leukaemia cells, Eur. J. Nucl. Med. Mol. Imaging 30(9):1251-61
- Bousis C, Emfietzoglou D, Nikjoo H (2012) Monte Carlo single-cell dosimetry of I-131, I-125 and I-123 for targeted radioimmunotherapy of B-cell lymphoma Int. J. Radiat. Biol. 88(12):908-915
1.11. Printed Paper:
Title: Photon albedo for water, concrete and iron at normal incidence, and dependence on the thickness of reflecting material
Authors: V.M. Markovic, D. Krstic, N. Stevanovic. and D. Nikezic
Journal: Nuclear Technology & Radiation Protection
ISSN: 1451-3994
Volume 28(1): 36-44, 2013, IF=1.159. (M22)
doi: 10.2298/NTRP1301000M
Category of Journal in year of publication and previous two years:
Year 2012: IF= 1.000, (M22)
Year 2011: IF= 1.159, (M22)
Year 2010: IF= 0.706, (M23)
Abstract
Total number and angular albedo were calculated for commonly used shielding materials, water, concrete and iron, for photons with initial energies from 10 keV up to 10 MeV and normal incident angle. Influence of material thickness on total number albedo also was investigated. Double differential albedo was determined from simulation of photon transport through materials by using PENELOPE and MCNP software. Backscattered photons were scored and grouped in equal intervals of energy and angle. Analytical expressions for angular and total number albedo as a function on initial energy were obtained. It was shown that angular albedo for three examined materials can be determined with the same formula. Corresponding analytical expressions for number albedos as a function of material thickness were presented in this paper.
References
[1] Pavlovic, R., Glodic, S., Boreli, F., Statistical weights as variance reduction method in back-scattered gamma radiation Monte Carlo spectrometry analysis of thickness gauge detector response. Prog. Nucl. Energ. (1990) 24 - 337
[2] Chilton, A.B., Shultis, J.K., Faw, R.E. Principles of Radiation Shielding. Prentice-Hall Inc., Englewood Cliffs, NJ, USA (1984)
[3] Shultis, J. K. Faw, R.E. Radiation Shielding. Prentice Hall PTR, Up per Saddle River, NJ, USA (1996)
[4] Bulatov, B. P., Efimenko, B. A., Zolotuhin,V. G., Klimanov, V. A. and Mashkovich V. P. Albedo of Gamma Radiation. Atomizdat, Moscow (1968)
[5] Ljubenov, V., Simovic, R., Markovic, S., Ilic, R., Number albedo of low-energy photons for water, aluminium and iron. Nucl. Tech. Rad. Prot. (2007) 22(1), 48-53
[6] Markovic V., Stevanovic N., Milenkovic B. and Nikezic D. Total number and angular albedo of photons for water, concrete and iron in dependence to material thickness 54. ETRAN conference, VI (2010)
[7] Krstic D., Markovic V. and Nikezic D. Reflection of electromagnetic radiation from shielding materials XXVI Symposium DZZSCG, 12.-14.X (2011)
[8] Krstic D., Markovic V.M., Nikezic D. and Stevanovic N. Total and angular photon albedo for water, concrete and iron, and dependence on the thickness of reflecting material. 14th International Congress of radiation Research, August 28 September 1, Warshaw, Poland (2011)
[9] Salvat, F., Fernández-Varea, J.M., Sempau, J., PENELOPE–2006 A Code System for Monte Carlo Simulation of Electron and Photon Transport. OECD Nuclear Energy Agency, Issy-les-Moulineaux, France (2006)
[10] X-5 Monte Carlo Team. MCNP–a General Monte Carlo N-Particle Transport Code, Version 5 Vol. I: Overview and Theory. Los Alamos, NM: Los Alamos National Laboratory; LA- UR- 03- 1987 (2003)
[11] Markovic, S., Lubenov, Lj., Simovic, R. and Ilic, R. Total number albedo of low energy photons, XXIV Symposium DZZSCG, 3-5.X (2007).
[12] Berger, M. and Raso, D. Monte Carlo calculation of gamma ray scattering. Rad. Res. (1960) 12, 20
[13] Ilic R. D. FOTELP-2K3, Photon, Electron, and Positron Monte Carlo Transport Simulation. IAEA 1388, OECD NEA Data Bank (2002)
[14] Bielajew A F, Rogers D WO (1987) PRESTA: The parameter reduced electron step transport algorithm for electron Monte Carlo transport. Nucl. Instrum. Meth. B 18, 165-181
[15] SIGMA PLOTÒ, Demo version of this software is available on web page http://www.systat.com/products/sigmaplot/
[16] Markovic S. Ljubenov V. Simovic R. A semiempirical formula for the angular diferential number albedo of low energy photons. Nucl. Tech. and Rad. Prot. (2005) 20(2), 28-34
[17] Markovic S. Rodoljub S. Ljubenov S. and Ilic D. R. Spectral albedos of photons of initial energies below 100 keV, Nucl. Techn. & Rad. Prot. (2007) 22(1), 40-47
[18] Ljubenov V. and Simovic R. Universal dependence of the total number albedo of photons on the mean number of photon scatterings, Nucl. Techn. & Rad. Prot. (2011) 26(3), 249-253
1.10. Printed Paper:
Title: Absorbed fractions in sensitive regions of human respiratory tract calculated by mcnp5/x software for electrons and beta particles due to radon progeny
Authors: D. Krstic, V.M. Markovic, D. Nikezic and D. Vucic.
Journal: Romanian Journal of Physics
ISSN: 1221-146X
Volume 58: S164–S171, 2013, IF= 0.745 (M23)
Category of Journal in year of publication and previous two years:
Year 2013: IF= 0.745, (M23)
Year 2012: IF= 0.526, (M23)
Year 2011: IF= 0.414, (M23)
Abstract
Radon, 222Rn, is radioactive noble gas which decays by alpha emission with half-life of 3.825 d. Its short-lived progeny, 218Po, 214Pb and 214Bi (214Po), are alpha and beta radioactive and they emit gamma radiation as well. Radon progeny can be inhaled by humans where they deposit on the inner layers of bronchi, and bronchioles. Particles (alpha, beta and gamma) emitted in radioactive decay damage surrounding tissue which can lead to development of lung cancer. The absorbed fractions (AF) of electrons and beta particles in sensitive layers of human respiratory tract were calculated in this paper. For that purpose the MCNP5/X simulation software [1], based on Monte Carlo method, was used. The human respiratory tract was modeled according to ICRP66 publication [2].
References
[1] X-5 Monte Carlo Team, MCNP–a General Monte Carlo N-Particle Transport Code, Version 5 Vol. I: Overview and Theory, Los Alamos, NM: Los Alamos National Laboratory; LA- UR- 03- 1987, 2003.
[2] ICRP Human respiratory model for radiological protection. A report of a task group the International Commission on radiological protection, ICRP Publication 66, Pergamon, Oxford, 1994.
[3] NCRP Report no. 93 Ionizing radiation exposure of the population of the United States. National Council on Radiation Protection and Measurements, Bethesda, Maryland, 1987.
[4] ICRP Limits for inhalation of radon daughters by workers. A Report of a Task Group the International Commission on Radiological Protection, ICRP Publication 32. Pergamon Press, Oxford, Vol 6, Issue 1, 1-24, 1981.
[5] Annals of the ICRP Guide for the Practical Application of the ICRP Human Respiratory Tract Model - ICRP Supporting Guidance 3Approved by ICRP Committee 2 in October 2000, Volume 32, Number 1, 13-14, 2002.
[6] Nelson WR, Hirayama H, Rogers DWO The EGS4 code system. Report SLAC-265, Stanford Linear Accelerator Center, Stanford, 1985.
[7] Fernández -Varea J M, Mayol R J, Baró, Salvat F On the theory and simulation of multiple elastic scattering of electrons. Nucl. Instrum. Meth. B 73, 447-473, 1993
[8] Markovic V, Stevanovic N, Nikezic D Absorbed fractions for electrons and beta particles in sensitive regions of human respiratory tract. Radiat Environ Biophys 47:139–145, 2008.
[9] Salvat F, Ferna´ndez-Varea JM, Sempau J PENELOPE–2006 a code system for monte carlo simulation of electron and photon transport. OECD Nuclear Energy Agency, Issy-les-Moulineaux, 2006.
[10] Markovic V M, Stevanovic N, Nikezic D. Doses from beta radiation in sensitive layers of human lung and dose conversion factors due to 222Rn/220Rn progeny, Radiat Environ Biophys, 50(3), 431-440, 2011.
1.9. Printed Paper:
Title: Influence electron motion in target atom on stopping power for low-energetic ions
Authors: N. Stevanovic. V.M. Markovic, M. Arsenijevic, D. Nikezic
Journal: Nuclear Technology & Radiation Protection
ISSN: 1451-3994
Volume 27(2): 113-116, 2012, IF=1.159 (M22)
doi: 10.2298/NTRP1202113S
Category of Journal in year of publication and previous two years:
Year 2012: IF= 1.000, (M22)
Year 2011: IF= 1.159, (M22)
Year 2010: IF= 0.706, (M23)
Abstract
References
1.8. Printed Paper:
Title: Doses from radon progeny as a source of external beta and gamma radiation
Authors: V.M. Markovic, D. Krstic, D. Nikezic, N. Stevanovic.
Journal: Radiation and Environmental Biophysics
ISSN: 0301-634X
Volume 51: 391–397, 2012, IF= 2.04 (M22)
doi: 10.1007/s00411-012-0413-1
Category of Journal in year of publication and previous two years:
Year 2012: IF= 1.754, (M22)
Year 2011: IF= 1.696, (M22)
Year 2010: IF= 2.040, (M22)
Abstract
Great deal of work has been devoted to determine doses from alpha particles emitted by 222Rn and its progeny. In contrast, contribution of beta particles and following gamma radiation to total dose has been neglected by most of the authors. The present work describes a study of the detriment of 222Rn progeny on the humans due to external exposure. Doses and dose conversion factor - DCF were determined for beta and gamma radiation in main organs and remainder tissue, taking into account 222Rn progeny 214Pb and 214Bi distributed in the atmosphere of room for Oak Ridge National Laboratory - ORNL phantom in the middle of the standard or typical room with dimensions 4´5´2.8m. DCF was found to be 7.37 μSv/WLM. Skin and muscle tissue from remainder receives largest dose. Beta and gamma doses from external exposure were compared with alpha, beta and gamma doses from internal exposure where source of radioactivity was lungs. Total doses received in all main organs and remainder tissues were obtained by summing up the doses from external and internal exposure and DCF was found to be 20.67 μSv/WLM.
References
Al-Jundi J, Li WB, Abusini M, Tschiersch J, Hoeschen C, Oeh U (2011) Inhalation dose assessment of indoor radon progeny using biokinetic and dosimetric modeling and its application to Jordanian population. Journal of Environmental Radioactivity 102:574-580
Amgarou K, Font L, Baixeras C (2003) A novel approach for long-term determination of indoor 222Rn progeny equilibrium factor using nuclear track detectors, Nuclear Instruments and Methods in Physics Research A, 506, 186–198
BEIR VI, Health Effects of Exposure to Radon: BEIR VI, Committee on Health Risks of Exposure to Radon, National Research Council, National academy press, Washington, D.C., 1999.
X-5 Monte Carlo Team. MCNP–a General Monte Carlo N-Particle Transport Code, Version 5 Vol. I: Overview and Theory. Los Alamos, NM: Los Alamos National Laboratory; LA- UR- 03- 1987; 2003.
Eatough JP, Henshaw DL (1992) Radon and thoron associated dose to the basal layer of the skin, Phys. Med. Biol. 37(4):955-67.
Eckerman KF, Cristy M, Ryman JC (1996) The ORNL mathematical phantom series. Available on http://ordose.ornl.gov/resources/Mird.pdf, last accessed on June 14, 2011.
ICRP (1981) Limits for inhalation of radon daughters by workers. A Report of a Task Group the International Commission on Radiological Protection, ICRP Publication 32. Pergamon Press, Oxford, Vol 6, Issue 1, 1-24.
ICRP 103 (2007) Quantities used in radiological protection. A Report of a Task Group the International Commission on Radiological Protection, ICRP Publication 103, Pergamon Press, Oxford, Annex B. 37: 247-322
Goorley T. MCNP Medical Physics Geometry Database. Los Alamos National Laboratory. Available at: http://mcnp-green.lanl.gov/publication/pdf/MP_DataBase_March2008-LA-UR-08-2113-release.pdf. Accessed 6 April 2011.
Jacobi W (1972) Activity and potential a energy of 222Rn and 220Rn daughters in different air atmosphere, Health Phys. 22, 441-450.
Kendall GM and Smith TJ (2002) Doses to organs and tissues from radon and its decay products J. Radiol. Prot. 22: 389–406
Krstic D Input files with ORNL-mathematical phantoms of the human body for MCNP-4b, Available on http://www.pmf.kg.ac.rs/radijacionafizika/InputFiles.html, last accessed on Accessed 6 April 2011.
Krstic D, Nikezic D (2007) Input files with ORNLmathematical phantoms of the human body for MCNP-4B. Comput. Phys. Commun. 176, 33–37.
Koblinger L (1978) Calculation of exposure rates from gamma sources in walls of dwelling rooms, Health Physics, 34, 459-463.
Koblinger L. (1984) Mathematical models of external gamma radiation and congruence of measurements, Radiation Protection Dosimetry, 7, 227-234.
Markkanen M (1995) Radiation Dose Assessments for Materials with Elevated Natural Radioactivity, STUK-B-STO 32, Finnish centre for radiation and nuclear safety, Helsinki.
Markovic VM, Krstic D, Nikezic D (2009) Gamma and beta doses in human organs due to radon progeny in human lung, Radiation Protection Dosimetry, 135 Issue: 3, 197 - 202.
NCRP Report no. 93 (1987) Ionizing radiation exposure of the population of the United States. National Council on Radiation Protection and Measurements, Bethesda, Maryland
Nikezic D. and Stevanovic N., 2007, Room model with three modal distributions of attached 220Rn progeny. Dose conversion factor for 220Rn progeny. Radiation Protection Dosimetry, 123, 95-102,
NRPB (1997) Assessment of skin doses, Documents of the NRPB: 8 (3)
NRPB (2000) Health Risks from Radon Faculty of Public Health Medicine, Chartered Institute of Environmental Health (Chilton: NRPB)
NRC (1999) Risk Assessment of Radon in Drinking Water (Washington, DC: National Academy Press)
Posner JD, Buchanan CR, Dunn-Rankin R (2003) Measurements and prediction of indoor air flow in a model room 35, 515-526.
Rosaline M, Mayya Y S, Kushwaha H S (2009) Measurement of 220Rn/222Rn progeny deposition velocities on surfaces and their comparison with theoretical models, Aerosol Science, 40, 1 – 15.
Table of Radioactive Isotopes, Periodic Table linked to decay data for known isotopes of each element. Available on http://ie.lbl.gov/education/isotopes.htm last accessed on February 17. 2011.
Tempfer H, Hofmann W, Schober A, Lettner H, Dinu AL (2010) Deposition of radon progeny on skin surfaces and resulting radiation doses in radon therapy, Radiat. Environ Biophys 49:249–259
Urosevic V, Nikezic D, Vulovic S (2008) A theoretical approach to indoor radon and thoron distribution, Journal of Environmental Radioactivity 99, 1829-1833.
UNSCEAR Report, Effects of ionizing radiation United Nations Scientific Committee, Annex E, 2006.
1.7. Printed Paper:
Title: Doses from beta radiation in sensitive layers of human lung and dose conversion factors due to 222Rn/220Rn progeny
Authors: V.M. Markovic, N. Stevanovic, D. Nikezic
Journal: Radiation and Environmental Biophysics
ISSN: 0301-634X
Volume 50(3), pp. 431-440, 2011, IF=2.04 (M22)
doi: 10.1007/s00411-011-0369-6
Category of Journal in year of publication and previous two years:
Year 2011: IF= 1.696, (M22)
Year 2010: IF= 2.040, (M22)
Year 2009: IF= 1.635, (M22)
Abstract
Great deal of work has been devoted to determine doses from alpha particles emitted from 222Rn and 220Rn progeny. Contribution of beta particles to total dose has been neglected by the most of the authors. A study of detriment of the 222Rn and 220Rn beta decaying progeny on a human lung was performed in this work. Doses and DCF were determined for beta radiation in sensitive layers of bronchi (BB) and bronchioles (bb), due to inhaled 222Rn and 220Rn progeny deposited in mucus and cilia layer. For that purpose electron absorbed fractions in sensitive layers of BB and bb regions were used. Activities in fast and slow mucus of BB and bb regions were obtained using LUNGDOSE software developed in earlier research. Calculated DCF due to beta radiation was 0.21 mSv/WLM for 222Rn and 0.06 mSv/WLM for 220Rn progeny. In addition influence of Jacoby room parameters on DCF was investigated and it was shown that DCF varies with changing parameters up to 50%.
References
Eisenbud M, Gesell T (1997) Environmental Radioactivity from Natural, Industrial, and Military Sources. San Diego, CA, Chapter 6 - Natural Radioactivity.
Fleischer R.L. (1997) Radon: Overview of properties, Origin and Transport. In: Durrani SA, Ilic R Radon measurements by etched track detectors. World Scientific, Singapore, 3, pp. 1-21
Darby S, Whitley E, Silcocks P, Thakrar B, Green M, Lomas P, Miles J, Reeves G, Fearn T, Doll R (1998) Risk of lung cancer associated with residential radon exposure in southwest England: a case–control study. Br. J. Cancer, 78, 394–408.
ICRP 32 (1981) Limits for inhalation of radon daughters by workers, Pergamon Press, Oxford, Vol 6, Issue 1, 1-24.
ICRP 66 (1994) Human Respiratory Model for Radiological Protection. Pergamon Press, Oxford, Vol 24 Issues 1-3: 1-482.
ICRP 103 (2007) Quantities used in radiological protection. Pergamon Press, Oxford, Anex B. 37: 247-322.
Jacobi W (1972) Activity and potential a energy of 222Rn and 220Rn daughters in different air atmosphere. Health Phys. 22, 441-450.
Jolyon HH, Simon LS, Wojcik A, Sohrabi M, Burkart W, Cardis E, Laurier D, Tirmarche M, Hayata I (2009) Human exposure to high natural background radiation: what can it teach us about radiation risks?. Journal of Radiological Protection, 29, А29-А42.
Markovic V, Stevanovic N, Nikezic D (2008) Absorbed fractions for electrons and beta particles in sensitive regions of human respiratory tract. Radiation and Environmental Biophysics, 47, 139–145.
NCRP Report no. 93 (1987) Ionizing radiation exposure of the population of the United States. National Council on Radiation Protection and Measurements, Bethesda, Maryland.
Nikezic D, Yu KN (2001) Microdosimetric calculations of absorption fraction and the resulting dose conversion factor for radon progeny. Radiation and Environmental Biophysics, 40, 207-211.
Nikezic D, Lau BMF, Stevanovic N, Yu KN (2006) Absorbed dose in target cell nuclei and dose conversion coefficient of radon progeny in the human lung. Journal of Environmental Radioactivity, 89, 18-29.
Nikezic D, Stevanovic N (2007) Room model with three modal distributions of attached 220Rn progeny and dose conversion factor. Radiation Protection Dosimetry, 123, 95-102.
Porstendorfer J (1991) Tutorial lessons : Properties and behavior of radon and thoron and their decay products in the air. In. IV Int. Symp. on the Nat. Radiat. Envir., Austria.
Porstendorfer J, Reineking A (2000) Radon characteristics related to dose for different living places of the human. Proceed. IRPA10, T-9-1. In: proceedings of the Tenth Congress of the International Radiation Protection Association, Hiroshima, Japan.
Ramachandran TV, Sahoo BK (2009) Thoron (220Rn) in the indoor environment and work places. Indian J. Phys. 83 (8): 1079-1098.
Steinhausler F (1996) Environmental 220Rn. A review. Environ. Intern., 22 (1), S1111-S1123.
UNESCAR Report (2000) Sources and effects of ionizing radiation. Volume I: Sources; Volume II: Effects, Unated Nations Scientific Committee on the Effect of Atomic Radiation, 2000 Report to General Assembly, with scientific Annexes. Unated Nations sales, Unated Nations, New York, Publication E.00.IX.3 and E.00.IX.4.
UNSCEAR Report (2006) Effects of ionizing radiation, United Nations Scientific Committee on the Effects of Atomic Radiation, UNSCEAR 2006 Report to the General Assembly, with scientific annexes. Unated Nations, New York, Volume II: Scientific Annex E.
Urosevic V, Nikezic D, Vulovic S (2008) A theoretical approach to indoor radon and thoron distribution. Journal of Environmental Radioactivity 99, 1829-1833.
Yamasaki T, Guo Q, Iida T (1995) Distributions of thoron progeny concentrations in dwellings. Radiat. Prot. Dosim. 59, 135-140.
Yu KN, Lau BMF, Nikezic D (2006) Assessment of environmental radon hazard using human respiratory tract models. Journal of Hazardous Materials, 132, 98-110.
Zhuo W, Iida T, Morizumi J, Aoyagi T, Takahashi I (2001) Simulation of the concentrations and distributions of indoor radon and thoron. Radiat. Prot. Dosim. 93, 357-368.
1.6. Printed Paper:
Title: Doses in human organs due to alpha, beta and gamma radiations emitted by thoron progeny in the lung
Authors: D. Nikezic, V.M. Markovic, D. Krstic and P.K.N. Yu.
Journal: Radiation Protection Dosimetry
ISSN: 0144-8420
Volume 141(4): 428-431, 2010, IF=0.966 (M22)
doi: 10.1093/rpd/ncq237
Category of Journal in year of publication and previous two years:
Year 2010: IF= 0.966, (M22)
Year 2009: IF= 0.707, (M23)
Year 2008: IF= 0.951, (M22)
Abstract
This work consists of two parts. In the first part, the doses in the human lung per unit exposure to thoron progeny, DCF, was calculated. Dependence of the DCF on various environmental and subject related parameters was investigated. The model used in these calculations was based on ICRP 66 recommendations. In the second part the human lungs were considered as the source of beta and gamma radiation which targets the other organs of the human body. The dose conversion factor to other organs (DCF) was obtained as 20 μSv/WLM which is larger than the DCF for radon progeny, which was 13 μSv/WLM. This is a consequence of the longer half life of relevant thoron progeny than that for radon progeny. It is interesting to note that after the lungs, where the radiation source is actually located, muscle tissue receives the largest dose.
References
- Steinhausler, F., Environmental 220Rn. A review. Environ. Intern., 22(1), S1111-S1123 (1996).
- Nikezic, D. and Yu, K. N., Microdosimetric calculations of absorption fraction and the resulting dose conversion factor for radon progeny. Radiat. Environ. Biophys. 40, 207–211 (2001).
- ICRP 66, Human Respiratory Model for Radiological Protection. A Report of a Task Group the International Commission on Radiological Protection. ICRP Publication 66 (Oxford: Pergamon Press) (1994).
- Nikezic D. and Stevanovic N. Room model with three modal distributions of attached 220Rn progeny and dose conversion factor. Rad.Prot.Dosim. 123, 95–102 (2007).
- ICRP 103, Quantities used in radiological protection. Pergamon Press, Oxford, Anex B. 37: 247-322 (2007).
- Eckerman, K. F., Cristy, M. and Ryman, J. C. The ORNL mathematical phantom series. Available on http://ordose.ornl.gov/, last accessed on April 26, 2010 (1996).
- Briesmeister, J. F. Ed. MCNP—a general Monte Carlo N-Particle Transport Code, Version 4B, LA-12625-M (New Mexico, Los Alamos: Los Alamos National Laboratory) (1997).
- Markovic, V. M., Krstic, D. and Nikezic, D., Gamma and beta doses in human organs due o radon progeny in human lung, Radiation Protection Dosimetry, 135 (3), 197 – 202 (2009).
- Marsh J.W. and Birchall A., Sensitivity analysis of the weighted equivalent lung dose per unit exposure from radon progeny, Radiat. Prot. Dosim, 87, 167-178 (2000).
1.5. Printed Paper:
Title: Relationship between deposition and attachment rates in the Jacobi room model
Authors: N. Stevanovic, V.M. Markovic, D. Nikezic.
Journal: Journal of Environmental Radioactivity
ISSN: 0265-931X
Volume 101 (5): 349-352, 2010, IF =1.466 (M23)
doi: 10.1016/j.jenvrad.2010.02.002
Category of Journal in year of publication and previous two years:
Year 2010: IF= 1.466, (M23)
Year 2009: IF= 1.268, (M23)
Year 2008: IF= 1.114, (M23)
Abstract
It is shown in this work that parameters of the Jacobi model, which describes behavior of short lived radon progeny, are not independent. The relationship between deposition rate of attached radon progeny and attachment rate of their unattached fraction was determined in this paper. It was found that deposition rate increases when the attachment rate is smaller; this effect is more pronounced for larger friction velocity. The deposition rate of attached radon progeny is presented here as a function of friction velocity, ventilation and attachment rate. Deposition rate of attached fraction was estimated in the range 0.012-0.46 h-1, when attachment rate varies from 10 h-1 to 100 h-1.
References
Amgarou, K., Font. L., Baixeras. C., 2003. A novel approach for long-term determination of indoor 222Rn progeny equilibrium factor using nuclear track detectors. Nucl Instrum. Meth. Phys. Res. A 506,186 –198.
International Commission on Radiological Protection. 1994. Human respiratory tract model for radiological protection. A report of a Task Group of the International Commission on Radiological Protection. Pergamon Press, Oxford.
Jacobi, W., 1972. Activity and potential a energy of 222Rn and 220Rn daughters in different air atmosphere. Health Phys. 22, 441– 450.
Knutson, E., George, A., Frey, J., Koh, B., 1983. Radon daughter plateout. Health Phys. 45, 445–452.
Lai, A. C. K., Nazaroff, W. W., 2000. Modeling indoor particle deposition from turbulent flow onto smooth surfaces. J. Aeros. Sci. 31(4), 463–476.
Marsh, J. W., Birchall, A., 2000. Sensitivity analysis of the weighted equivalent lung dose per unit exposure from radon progeny. Radiat. Prot. Dosim. 87, 167-178.
MATHEMATICA®. Available on the web page http://wolfram.com
Mishra, R.S., Mayya, Y.S., Kushwaha, H.S., 2009. Measurement of 220Rn/222Rn progeny deposition velocities on surfaces and their comparison with theoretical models. J. Aer. Sci. 40, 1-15.
Stevanovic, N., Markovic V. M., Urosevic, V., Nikezic, D. 2009a. Determination of parameters of the Jacobi room model using the Brownian motion model. Health Phys. 96(1), 48-54.
Stevanovic, N., Markovic, V.M., Nikezic, D. 2009b Deposition rates of unattached and attached radon progeny in room with turbulent airflow and ventilation. J. Envi. Rad. 100, 585–589.
Porstendörfer, J., 1984. Behaviour of radon daughter products in indoor air. Radiat. Prot. Dosim. 7, 107–113.
Porstendörfer, J., 1994., Properties and behaviour of radon and thoron and their decay products in the air. J. Aerosol Sci. 25 (2), 219-263, 1994.
Zhao, B., Wu, J., 2006. Modeling particle deposition from fully developed turbulent flow in ventilation duct. Atmos. Envir. 40, 457–466.
1.4. Printed Paper:
Title: Gamma and beta doses in human organs due to radon progeny in human lung
Authors: V.M. Markovic, D. Krstic, D. Nikezic
Journal: Radiation Protection Dosimetry
ISSN: 0144-8420,
Volume 135(3): 197 – 202, 2009, IF= 0.951 (M22)
doi: 10.1093/rpd/ncp106
Category of Journal in year of publication and previous two years:
Year 2009: IF= 0.707, (M23)
Year 2008: IF= 0.951, (M22)
Year 2007: IF= 0.528, (M23)
Abstract
A great deal of work has been devoted to determine the effect of tissue damage produced by alpha particles emitted from radon and its progeny. 214Pb and 214Bi deposited in human lung emit beta particles followed by the gamma quanta, which cause smaller damage of tissue in comparison with alpha particles. Because of that, this type of irradiation has not been studied in details. In this paper, doses from beta and gamma rays emitted by radon progeny 214Pb and 214Bi in lung has been calculated in all main organs and remainder tissue of human body. Human ORNL phantom of adult male and female was used, where simulation was performed using MCNP-4B simulation code. The source of beta and gamma radiation, namely, radon progeny were placed in lungs. Furthermore, dose conversion coefficients have been calculated.
References
- ICRP, Human Respiratory Model for Radiological Protection. A Report of a Task Group the International Commission on Radiological Protection. ICRP Publication 66. Pergamon Press, Oxford (1994).
- Marsh, J.W. Bessa, Y. Birchall, A. Blanchardon, E. Hofmann, W. Nosske D. and Tomasek, L. Dosimetric models used in the alpha-risk project to quantify exposure of uranium miners to radon gas and its progeny. Radiation Protection Dosimetry, 130, 101-106 (2008).
- Nikezic D. and Yu, K.N. Microdosimetric calculations of absorption fraction and the resulting dose conversion factor for radon progeny. Radiat. Environ. Biophys. 40, 207-211 (2001).
- Nikezic, D. Lau, B.M.F. Stevanovic, N. Yu, K.N. Absorbed dose in target cell nuclei and dose conversion coefficient of radon progeny in the human lung. Journal of Environmental Radioactivity, 89, 18-29 (2006).
- Krstic, D., Nikezic, D. Input files with ORNL-mathematical phantoms of the human body for MCNP-4B. Computer Physics communications 176, 33-37 (2007).
- Briesmeister, JF (Ed). MCNP - A General Monte Carlo N- Particle Transport Code, Version 4B, LA-12625-M. Los Alamos National Laboratory, Los Alamos, New Mexico (1997).
- ICRP 60. International Commission on Radiological Protection. Recommendations of the International Commission on Radiological Protection. Oxford: Pergamon Press, ICRP Publication 60 (1991).
- Eckerman, K.F. Cristy, M. and Ryman, J. C. The ORNL mathematical phantom series, available at: http://homer.ornl.gov/vlab/mird2.pdf (1996).
- Input files with ORNL-mathematical phantoms of the human body for MCNP-4b, available at: http://www.pmf.kg.ac.yu/radijacionafizika/InputFiles.html.
- http://ie.lbl.gov/education/isotopes.htm Table of Radioactive Isotopes. Periodic Table linked to decay data for known isotopes of each element.
- Markovic, V. Stevanovic, N. and Nikezic, D. Absorbed fractions for electrons and beta particles in sensitive regions of human respiratory tract. Radiation and Environmental Biophysics. 47, 139–145 (2007).
- Marsh, JW. Birchall, A. Sensitivity analysis of the weighted equivalent lung dose per unit exposure from radon progeny. Radiat Prot Dosim 87, 167–178 (2000).
- ICRP 74. International Commission on Radiological Protection. Conversion factors for use in radiological protection against external radiation. Oxford: Pergamon Press, ICRP Publication 74 (1996).
- ICRP 103. International Commission on Radiological Protection. Anex B. Quantities used in radiological protection. Vol 37, 247-322 (2007)
1.3. Printed Paper:
Title: Deposition rates of unattached and attached fractions in room at turbulent airflow and ventilation
Authors: N. Stevanovic V.M. Markovic, D. Nikezic.
Journal: Journal of Environmental Radioactivity
ISSN:0265-931x,
Volume 100(7): 585-589, 2009, IF =1.268, (M23)
doi: 10.1016/j.jenvrad.2009.04.007
Category of Journal in year of publication and previous two years:
Year 2009: IF= 1.268, (M23)
Year 2008: IF= 1.114, (M23)
Year 2007: IF= 0.963, (M23)
Abstract
In this paper deposition rate coefficients for unattached and attached radon progeny were estimated according to a particle deposition model for turbulent indoor airflow described by Zhao and Wu [2006. Modeling particle deposition from fully developed turbulent flow in ventilation duct. Atmos. Environ. 40, 457-466]. The parameter which characterizes turbulent indoor airflow in this model is friction velocity, u*. Indoor ventilation changes indoor airflow and friction velocity and influences deposition rate coefficients. Correlation between deposition and ventilation rate coefficients in the room was determined. It was shown that deposition rate coefficient increases with ventilation rate coefficient and that these parameters of the Jacobi room model cannot be assumed to be independent. The values of deposition rate coefficients were presented as functions of friction velocity and ventilation rate coefficient. If ventilation rate coefficient varies from 0.1 up to 1h(-1), deposition rate coefficients for unattached and attached fractions were estimated to be in the range 3-110 h(-1) and 0.015-0.35 h(-1), respectively.
References
Amgarou, K., Font. L., Baixeras. C., 2003. A novel approach for long-term determination of indoor 222Rn progeny equilibrium factor using nuclear track detectors. Nucl Instrum. Meth. Phys. Res. A 506,186 –198.
International Commission on Radiological Protection. 1994. Human respiratory tract model for radiological protection. A report of a Task Group of the International Commission on Radiological Protection. Pergamon Press, Oxford.
Jacobi, W., 1972. Activity and potential a energy of 222Rn and 220Rn daughters in different air atmosphere. Health Phys. 22, 441– 450.
Knutson, E., George, A., Frey, J., Koh, B., 1983. Radon daughter plateout. Health Phys.45, 445–452.
Lai, A. C. K., Nazaroff, W. W., 2000. Modeling indoor particle deposition from turbulent flow onto smooth surfaces. J. Aeros. Sci. 31(4), 463–476.
Marsh, J. W., Birchall, A., 2000. Sensitivity analysis of the weighted equivalent lung dose per unit exposure from radon progeny. Radiat. Prot. Dosim. 87, 167-178.
Marsh, J. W., Birchall, A., Butterweck, G., Dorrian, M. D., Huet, C., Ortega, X., Reineking, A., Tymen, G., Schuler, Ch., Vargas, A., Vezzu, G., Wendt, J., 2002. Uncertainty analysis of the weighted equivalent lung dose per unit exposure to radon progeny in the home. Radiat. Prot. Dosim. 102, 229-248.
Porstendörfer, J., 1984. Behaviour of radon daughter products in indoor air. Radiat. Prot. Dosim. 7, 107–113.
Reineking, A., Knutson, E. A., George, A. C., Solomon, S. B., Kesten, J., Butterweck, G., PorstendEorfer, J., 1994. Size distribution of unattached and aerosol-attached short-lived radon decay products: Some results of inter-comparison measurements. Radiat. Prot. Dosim. 56(1–4), 113–118.
Straw, P.M., 2000. Computation and Measurement of Wind Induced Ventilation. Doctoral dissertation , University of Nottingham.
Stevanovic, N., Markovi,c V. M., Urosevic, V., Nikezic, D. 2009. Determination of parameters of the Jacobi room model using the Brownian motion model. Health Phys. 96(1), 48-54.
Zhao, B., Wu, J., 2006. Modeling particle deposition from fully developed turbulent flow in ventilation duct. Atmos. Envir. 40, 457–466.
Zhuo, W., Iida, T., Moriizumi, J., Aoyagi, T., Takahashi, I. 2001. Simulation of the concentrations and distributions of indoor radon and thoron. Radiat. Prot. Dosim. 93 (4), 357–368.
1.2. Printed Paper:
Title: Determination of parameters of Jacobi room model using the Brownian motion model
Authors: N. Stevanovic V. Markovic, V. Urosevic and D. Nikezic
Journal: Health Physics
ISSN: 0017-9078
Volume 96(1): 48-54, 2009, IF=0.917, (M22)
doi: 10.1097/01.HP.0000326328.47540.6d
Category of Journal in year of publication and previous two years:
Year 2009: IF= 0.917, (M22)
Year 2008: IF= 0.869, (M22)
Year 2007: IF= 0.870, (M22)
Abstract
Parameters of the Jacobi room model were estimated with simulation of Brownian motion. Deposition on internal room surfaces and attachment of progeny atoms to three modally distributed aerosols were taken into account. The values of parameters were presented as functions of aerosol concentrations.
The deposition rate of an unattached progeny was estimated in the range 30-47 h-1; the deposition rate of an attached progeny was very small and its range is 0.0007-0.004 h-1; the attachment rate of a progeny is in range 40-170 h-1. The statistical uncertainty was lower than 1%. The ranges of parameters were similar to those reported in literature.
References
Amgarou K, Font L, Baixeras C. A novel approach for long-term determination of indoor 222Rn progeny equilibrium factor using nuclear track detectors. Nucl. Instrum. Meth. Phys. Res. A 506:186-198; 2003.
Darby S, Whitley E, Silcocks P, Thakrar B, Green M, Lomas P, Miles J, Reeves G, Fearn T, Doll R. Risk of lung cancer associated with residential radon exposure in southwest England: a case-control study. British Journal of Cancer 78: 394-408; 1998.
Hinds WC, Aerosol Technology, Properties, Behavior and Measurement of Airborne Particles, Wiley & Sons, New York, 1998.
Jacobi W, Activity and potential a energy of 222Rn and 220Rn daughters in different air atmosphere. Health Phys 22:441-450; 1972.
Landau LD, Lifshic EM. Mechanic of Continuum Media, second ed., by Gradjevinska knjiga, 1965 (in Serbian-translation from Russian).
Marsh JW, Birchall A. Sensitivity analysis of the weighted equivalent lung dose per unit exposure from radon progeny. Radiat Prot Dosim 87:167-178; 2000.
Nazaroff WW, Nero AV. Radon and Its Decay Products in Indoor Air. John Wiley and Sons, New York, 1988.
Nikezic, D, Stevanovic N. Room model with three modal distributions of attached radon progeny. Health Phys. 87(4):405-409; 2004.
Nikezic D, Stevanovic N. Radon progeny behavior in diffusion chamber. Nucl. Instrum Meth B 239: 399-406; 2005.
Nikezic D, Stevanovic N. Behavior of 220Rn progeny in diffusion chamber. Nucl. Instrum Meth Phys Res A 570: 182–186; 2007.
Nikolopoulos D, Vogiannis E. Modeling radon progeny concentration variations in thermal spas. Science of the Total Environment 373: 82–93; 2007.
Porstendörfer J. Physical parameters and dose factors of the radon and thoron decay products. Radiat Prot Dosim 94(4): 365–73; 2001.
Reist PC, Aerosol Science and Technology, second ed., McGraw-Hill, New York, 1993.
Stevanovic N, Nikezic D, Djordjevich A. The recoil factor of 214Pb. Journal of Aerosol Science 35: 1041-1050; 2004.
Zhuo W , Iida T, Moriizumi J, Aoyagi T, Takahashi I. Simulation of the concentrations and distributions of indoor radon and thoron. Radiat Prot Dosim 93(4): 357-368; 2001.
1.1. Printed Paper:
Title: Absorbed fractions for electrons and beta particles in sensitive regions of human respiratory tract
Authors: V. Markovic, N. Stevanovic and D. Nikezic
Journal: Radiation and Environmental Biophysics
ISSN: 0301-634X
Volume 47:139-145, 2008, IF=1.787, (M22)
doi: 10.1007/s00411-007-0135-y
Category of Journal in year of publication and previous two years:
Year 2008: IF=1.787, (M22)
Year 2007: IF=1.070, (M23)
Year 2006: IF=1.090, (M23)
Abstract
The absorbed fractions (AF) of electrons in sensitive layers of human respiratory tract were calculated in this paper. For that purpose the source code for simulation package PENELOPE, based on Monte Carlo method, was developed. The human respiratory tract was modeled according to ICRP66 publication, where AF of electrons was calculated using EGS4 simulation software. Some approximations used in ICRP66 were corrected in this work, and new values of AF for radon progeny are given. Minimal energy (EABS) that electron can have during transport through material is 1 keV in ICRP66, while it is set as low as 100 eV in the presented work. Lowering value of EABS gives more accurate results for AF when initial energy of electrons is below 50 keV. To represent tissue, water is used in ICRP66, while in this work epithelia tissue is used.
References
- Darby S, Whitley E, Silcocks P, Thakrar B, Green M, Lomas P, Miles J, Reeves G, Fearn T, Doll R (1998) Risk of lung cancer associated with residential radon exposure in southwest England: a case-control study. British Journal of Cancer 78, 394-408
- ICRP (1994) Human Respiratory Model for Radiological Protection. A Report of a Task Group the International Commission on Radiological Protection, ICRP Publication 66. Pergamon Press, Oxford
- Annals of the ICRP (2002) Guide for the Practical Application of the ICRP Human Respiratory Tract Model - ICRP Supporting Guidance 3Approved by ICRP Committee 2 in October 2000, Volume 32, Number 1, 13-14
- Nelson W R, Hirayama H, Rogers D W O (1985) The EGS4 Code System. Report SLAC-265. Stanford Linear Accelerator Center, Stanford, California, US.
- Bielajew A F, Rogers D WO (1987) PRESTA: The parameter reduced electron step transport algorithm for electron Monte Carlo transport. Nucl. Instrum. Meth. B 18, 165-181
- Molière G (1948) Theorie der Streuung schneller geladener Teilchen II. Mehrfachund Vielfachstreuung. Z. Naturforsch. 3, 78-97
- Fernández -Varea J M, Mayol R J, Baró, Salvat F (1993) On the theory and simulation of multiple elastic scattering of electrons. Nucl. Instrum. Meth. B 73, 447-473
- Salvat F, Fernández-Varea J M, Sempau J (2003) PENELOPE – A Code System for Monte Carlo Simulation of Electron and Photon Transport. Workshop Proceedings. Issy-les-Moulineaux, France
- Ziegler J F, Biersack J P, Littmark U (1985) The Stopping and Range of Ion in Matter. Pergamon Press. Calculations were performed with SRIM-2003 (see http://www.SRIM.org)
|
 |